By François Lempérière and Luc Deroo
Almost all countries have built dams across rivers, mainly for supplying hydropower or storing water. The reservoirs are filled by gravity. The average per capita world hydropower supply is about 500 kilowatt-hours (kWh) per year, making up about 10 percent of the total use of energy. The average per capita useful storage of drinkable or irrigation water worldwide is about 100 cubic meters (m3), or 3,531 cubic feet (ft3). That figure is being reduced by the siltation of reservoirs.
The global need for power and water is increasing dramatically, but traditional dam construction is now much reduced in most countries for three reasons: Most of the best sites in rivers for traditional dams are already taken; the direct costs of wind and photovoltaic (PV) energy are now lower than those of hydropower; and there is increasing criticism of the environmental impact of dams, especially the 500,000 square kilometers (km2), or 19,305 square miles (mi2), of drowned areas along rivers. If current situations continue, these factors may significantly reduce the rate of traditional dam construction within 20 years. However, there is also the possibility that innovations in the use of dams may increase the rate of dam construction and launch a new golden age for dams. One key innovation may be the use of pumping to fill reservoirs for purposes including energy, water storage, and flood mitigation.
The Need for Pumped Storage Facilities
The need for electric energy storage is presently low, because most electricity is generated by flexible coal or gas thermal plants. However, the need will be high within a few decades, because most energy will probably be electricity from intermittent wind and solar PV installations. The storage need will be especially important in the sunny countries located on average around 1,500 km (932 mi) from the Equator, which are currently home to 5 billion people and will have 7 or 8 billion in 2050. The revenue per capita and use of energy per capita of these countries in Africa, South Asia, and Latin America are only 20 percent of those in the United States and Europe.
For centuries, the sunny countries had fewer easily used energy sources than Europe, which may be a key reason for their lower economic progress. The future availability throughout the year of unlimited PV energy in sunny countries at a direct cost of $20–30 per megawatt-hour (MWh), however, is a miracle that should be quickly taken advantage of, but which requires a huge amount of energy storage to store energy generated during the day for use at night. An increase in energy production from the current level of 8,000 terawatt-hours (TWh) for 5 billion residents to a potential future level of 50,000 TWh for 8 billion residents could be the engine of enormous economic progress. The figure of 50,000 TWh would be based on 40,000 TWh of low-cost PV generation. One half of that generation would be used directly during the 10 hours of sunlight per day, but most needs beyond daytime will rely on stored energy, which would be stored primarily by pumped storage plants. Assuming that these pumped storage plants would need to store 10,000 TWh of energy over 3,000 hours, we are looking at a needed storage capacity of 3,000–4,000 gigawatts (GW). During peak times, electricity storage needs may also be met with batteries, but pumped storage is probably a better solution for daily storage: The investment by stored kWh would not be too different, but the life of batteries can be counted in years, while the life of pumped storage projects may last centuries.
The 1.3 billion people who live in the countries of the Organisation for Economic Co-operation and Development (OECD), mainly in North America and Europe, live on average 5,000 km (3,107 mi) from the Equator and have fewer hours of sun but much more wind than the sunny countries. They presently use 10,000 TWh a year of electricity, mostly thermally generated, although with about 20 percent generated by nuclear facilities and about 15 percent by hydropower. The population of these countries is not increasing, so their total energy use may not increase much, but the energy share of electricity will likely increase, possibly up to about 15,000 TWh a year, including 2,500 TWh of hydro, perhaps a few thousand thousand TWh of nuclear, little fossil energy, offshore and onshore wind power, and some PV energy during the sunny parts of the year. Wind generation may vary day by day, but spreading windmills across a large area will reduce the OECD nations’ storage needs to around 1,000 GW.
For 40 years, China has increased its revenue per capita tenfold, thanks to an increase of electricity per capita from almost nothing to 6,000 kWh for 1.3 billion people, mainly produced with coal. In the future, China will use mainly wind and PV energy and its population, energy use, and need for energy storage may approach those of the OECD nations.
All told, the world need for pump storage plants may surpass 5,000 GW, which will need to be implemented from 2030 to 2080, i.e., at a rate of over 100 GW a year. The rate of investment in traditional hydropower has only been 20 GW a year for the past 70 years, and the cost of dams per GW is not much different. The necessary yearly investment in dams for electric energy storage in 2040 may thus be five times the investment in hydropower during the golden age of dams around 1970. The likely increase in the total world revenue in 70 years is also about fivefold.
How Can 5,000 GW of Pumped Storage Plants Be Built?
The traditional solution that has been employed in the 200 GW worth of existing pumped storage plants involves using tunnels to link two reservoirs whose level differs by several hundred meters. Using mountainous terrain where the level differs even more has huge extra potential, but this solution has two drawbacks: The use of rather long tunnels prevents a quick adjustment in plant operations to respond to quick changes in PV or wind availability, and this solution can only be implemented in rather steep areas in the upper parts of large catchment areas. However, there are two other pumped storage solutions with significant potential: twin dams along rivers and pumped storage plants along sea cliffs.
Twin Dams
A typical example of a large traditional hydropower scheme is a 100-m-high (328-ft-high) dam creating a 20-km-long (12.4-mi-long) reservoir on a river. Throughout most of the year, the power plant uses most of the river flow under a head close to 100 m (328 ft). An alternative is the use of two 50-m-high (164-ft-high) dams, creating two nearby 10-km-long (6.2-mi-long) reservoirs and using two plants under a 50-m (164-ft) head for a power supply similar to that of the basic solution. In the latter case, it may be possible to use a pumped storage plant to exchange water between the two reservoirs on a daily basis (fig. 1). The water volume pumped yearly may be 10–50 times the yearly river flow, and while it is used under a head of 50 m instead of 100 m, the yearly pumped energy may be 5–20 times the supplied power. After 2030, this may be a cost-effective solution for supplying full-time intermittent solar or wind energy in many countries. A significant quantity of PV elements may be set on the reservoirs themselves. This twin dams solution should be studied for most new large schemes; it may also replace an existing reservoir, add a new reservoir upstream or downstream of an existing reservoir, or associate a reservoir in a main river with a higher reservoir in a tributary.
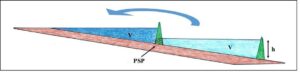
The 5,000-km2 (1,930-mi2) Kariba Reservoir in Africa, which supplies 7 TWh a year, could be adapted to store over 200 TWh a year (fig. 2). Along similar lines, the 300-km-long Aswan Reservoir in southern Egypt, which has a useful water storage of 100 billion m3 (81 million acre-feet) and supplies 7 TWh a year of hydropower, could be divided into two reservoirs and supplemented with a 100-km-long (62-mi-long) reservoir in an old tributary that is now fully dry. The Aswan Reservoir’s huge water storage capacity could be preserved while simultaneously allowing it to store 500 TWh a year of low-cost PV energy generated in a region where there are never clouds (fig. 3). Egypt could have enough low-cost energy for all its needs and to export to Southern Europe or the Middle East. This Aswan scheme could be implemented over 50 years in 10 steps of 20 GW each. This is the largest possible pumped storage scheme; the usual capacity of most projected twin dams schemes is 1–10 GW.
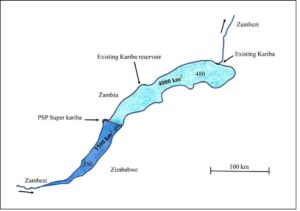
Sea Cliff Pumped Storage Projects
Worldwide, there are over 100,000 km (62,137 mi) of seaside cliffs. Usually, the sea within 1–2 km (0.62–1.24 mi) of the cliff toe is not particularly deep, and the seabed is rock for several hundred meters and thus favorable to pumped storage plant construction. Building 100-m-high (328-ft-high) dams along the seacoast could be used to create high reservoirs along cliffs, using the ocean itself as the low reservoir. This solution is too expensive for small capacities, but appears to be cost effective for schemes of at least several GW. This concept can also be adapted for very large natural lakes. In many sites, the high reservoir may be partly or totally above the cliff, thus reducing its cost per GW. The construction of the dam at sea may occur behind a rockfill breakwater, which can be used later as the downstream toe of the dam. Construction in calm water may be easier than it was for many existing dams in large rivers (fig. 4). The potential for pumped storage projects on islands is lower, as the cost per kWh would usually be higher.
The Cost of Pumped Storage and of Future World Electricity
Most future world energy is likely to be electricity, primarily supplied by PV and windmills, with about 10 percent supplied by flexible hydropower supply. After 2050, the direct cost of PV will be 2–3 cents per kWh in sunny countries and 4–5 cents elsewhere. The direct cost of wind power and hydropower will be on average under 5 cents per kWh. The average direct cost of electricity will thus probably be 3–4 cents per kWh in sunny countries and about 5 cents elsewhere.
Thirty or forty percent of that energy will be stored, mainly through pumped storage, with a loss of about 25–40 percent, i.e., an increase of 10 percent of the direct cost. The cost of storage by pumped storage projects derives mainly from the initial investment; their total yearly cost may be 6–7 percent of this investment. The investment for future pumped storage facilities will usually be $1,000– $1,500 per kW. The cost per kW for the pump turbines will likely be reduced by the huge quantity of similar equipment that will be produced worldwide each year. These costs are high for hydropower today because a scheme requires a small number of units of a specific design. The yearly cost per kW of a pumped storage plant will likely be 6–7 percent of $1,000–$1,500—that is, $60–$100 for 2,500 hours of pumping, or 3–4 cents per stored kWh or about 1 cent per kWh used. Adding the cost of some additional battery or hydrogen storage and storage losses, the total future cost of electricity per kWh may be about the direct cost of PV or wind power plus 2 cents.
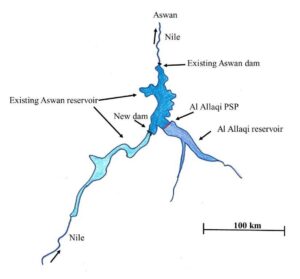
The Promise of Large, Low Off-River Dams
The total world capacity of reservoirs created by dams is over 7,000 km3 (5.67 billion acre-feet), but most is devoted to hydropower. The capacity devoted to seasonal water storage is reduced by siltation and evaporation to under 1,000 km3 (810.7 million acre-feet); that is, about 100 m3 (3,531 ft3) per capita for the world’s 8 billion people. Today, billions of people lack water storage, especially in sunny countries, and considering population increase and the increase in food quality, the world’s future needs for seasonal water storage could double within 50 years.
Traditional water storage by dams on rivers requires a minimal river slope and does not apply to rather flat areas where storing water off rivers by pumping may be a cost-efficient alternative. The cost of low-head pumping is low; most of the cost is for the dikes surrounding reservoirs. The cost per cubic meter of stored water may be acceptable for reservoirs with a surface area of 1–10 km3 (0.38–3.8 mi3) or more and with an average depth of 5–10 m (16.4– 32.8 ft). Surprisingly, this is close to or greater than the average depth of existing dammed reservoirs devoted to water storage, which is about 20 percent of the dam height. Fifty percent of existing large water storage dams are less than 30 m (98.4 ft) tall, and there are many hundreds of thousands of small dams under 15 m (49.2 ft) tall. This solution has great potential and could be used to meet a huge part of future needs. An off-river reservoir of this type may be close to the river or within a few kilometers of it and could be a dozen meters above it. Off-river reservoirs filled by pumping have been used for over a century, but usually for rather small volumes and thus at a much higher cost per cubic meter than would be the case with the proposed large reservoirs. Off-river reservoirs would also be more environmentally friendly that traditional on-river reservoirs.
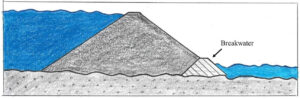
Large off-river dams can also serve for flood mitigation. Today, there are many fewer fatalities from floods than in the past, thanks to flood forecasting and efficient communications, but property damage is likely to increase along with population, home values, and climate change. Using on-river dams to store floodwater is efficient in steep portions of rivers, but not in flat areas where many people live close to the river. Using pumping to fill large, off-river reservoirs for water storage may be cost efficient. It would require large pumping capacity, but the power needs would be limited by the low head. Such reservoirs may also be used for seasonal water storage. It may be difficult to find a reservoir with a surface area of dozens of square kilometers in the downstream, populated sections of large rivers, but several smaller reservoirs on tributaries may be an efficient alternative.
Increasing the Water Speed of Large Rivers
In the downstream sections of large rivers, the slope is usually less than 0.5 m/km (2.64 ft/mi), the water depth 5–10 m (16.4–32.8 ft), and the water speed during extreme floods about 2 m (6.5 ft) per second. Increasing the water speed by 10–20 percent would reduce the water level by 0.5–1 m (1.6–3.2 ft) and thus avoid most damage. If a dam on a river downstream of a large city is paired with a pumping plant that pumps river flow upstream to downstream during a flood, it could reduce the water level by 2 m (6.4 ft) close to the dam and by an average of 1 m (3.2 ft) over 10–20 km (6.2–12.4 mi), avoiding most damage. This solution seems cost effective for rivers with a slope under 0.5 m/km (2.64 ft/mi). In terms of power, it would likely require several dozen MW. This solution may also be used in large deltas. Examples of locations where this solution may be used include Paris, Bangkok, and the Mekong Delta, where tens of millions of people live.
Environmental Effects
The environmental effects of the innovative uses described above are much better than those of traditional dams. For example,
• Off-river water storage avoids the effects on rivers that traditional dams have.
• Twin dam reservoirs of the type described above occupy one-tenth of the surface area per GW that traditional dammed reservoirs do.
• Pumped storage plants along the sea have comparative lower effects per GW.
Most criticisms of PV and wind energy focus on the need to store intermittently generated energies, especially on the surface areas of the pumped storage reservoirs that would be necessary. However, media presentations by experts on these topics often refer to the surface area per GW of existing reservoirs supplying hydropower— on average, 300 km2 (115.8 mi2) per GW. Actually, the surface area needed for pumped storage reservoirs is only about 10 km2 (3.8 mi2) per GW. Five thousand GW of pumped storage will require 50,000 km2 (19,305 mi2), 10 percent of the present reservoir area for 1,300 GW of hydropower supply.
Future Investments in Dams
The investment in future energy generation and storage dams may include 500 GW of traditional hydropower supply, 200 GW of tidal plants, and 5,000 GW of pumped storage plants. Investments for water storage may reach 1 trillion m3, much of which could be off-river storage. Flood mitigation needs may be met by traditional solutions in the upstream sections of rivers and by pumping in the downstream sections, either by storage or by water speed increase. Most needs and investments will be in the sunny countries within 1,500 km (932 mi) of the Equator. The innovative uses we have described here are generally based on traditional dam design and construction methods, but they may be significantly more cost effective. These innovative solutions are also less susceptible to the main cause of failure of traditional dams, namely exceptional floods. There is a bright future for the construction of new, innovative dams, especially large, environmentally friendly pumping schemes of the type that will be in great demand in future decades in the world’s sunny countries.
François Lempérière is the president of HydroCoop.
Luc Deroo is the managing director of ISL. For more about ISL, visit www.isl.fr/en.